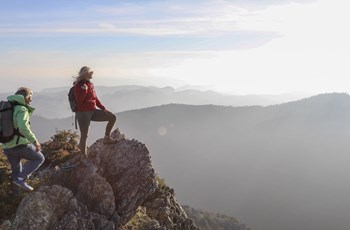
Mental health is on all of our minds, now more than ever after the COVID pandemic! The acuteness of stress in everyday life, even before the start of the pandemic, brought out the necessity to reshape how we understand and approach mental health. The previously ingrained notion that mental health (or lack thereof) is solely a brain-only problem ought to be dispelled [1].
Don’t get me wrong, the brain is truly magnificent in its complexity, orchestrating the delicate interplay between the body and the mind. All the sensory information—anything the body senses, feels, hears, smells, touches, or ingests—this plethora of inputs engages the brain to interpret and stimulate our feelings, thoughts, reactions, and behaviour. The brain is a powerful master controller, the seat of memory, intelligence, and a myriad of cognitive processes; it is the true source of all the things that define our humanness. However, viewing it separate from the rest of the body is inherently limiting, especially in the context of mental health, as this approach desensitizes us to the influences of our lifestyle, environment, genetics, and prior health history [2].
After all, the brain and the body are interconnected in the most marvelous and elaborate ways that rely on the rich bi-directional communication network to allow the individual to thrive. And when one of the system’s components falls out of sync with the other, that delicate balance is lost, supporting favourable conditions for disease to plant its roots. Conceptually, this interconnectedness is not a novel paradigm, but rather an attempt to rationalize and explain disorders pertaining to brain, and therefore mental health from the systems biology angle.
Urinary Neurotransmitter Testing
The neurotransmitter test provides a framework for understanding the connection of our body’s physical health to our brain’s well-being. In a nutshell, it is an effective advanced screening tool designed to gather information about the levels of specific neurotransmitters, amino acid precursors, and metabolites originating in various organs throughout the body. These molecules convey messages to the brain either directly by crossing the blood-brain barrier (BBB), indirectly via the nerves of the enteric nervous system, or the vagus nerve itself [3]. The levels of these molecules, determined by the neurotransmitter test (too low, too high, or just right, i.e., within range), are as individual as each individual patient, reflective of that person’s own ecosystem (genes + lifestyle + environment).
Discovering the detailed interplay between neurotransmitters and perhaps other endocrine signalling molecules, has helped fortify our understanding of how biological function in the body influences brain health. The results of the test provide a biochemical basis that helps explain mental health-related symptoms the patient is suffering from and enable the practitioner to move forward with creating a highly individualized therapeutic intervention, usually based on a combination of lifestyle and dietary changes that include personalized nutritional intervention strategies (e.g., methylation support, antioxidants, targeted amino acids, vitamins + minerals, adaptogens, etc.).
This test is a great example of how complementary medicine tackles the notion of “chemical imbalance” from something hypothetical, subjective, and rather elusive; to quantifiable, objective and, more importantly, therapeutically actionable.
Expanding the Neuro Advanced Profile
During the past year, we have accelerated our efforts to combine our vast laboratory testing experience with current clinical literature findings to advance the current offerings in our Neurotransmitter test. The newly expanded profile now offers 11 additional analytes that complement the previous panel to offer an even more focused assessment of biological function of the brain and the body.
Taurine
One such new analyte that has prominently taken residence in ZRT’s Neurotransmitter test, is the sulfur-containing amino acid taurine. Taurine, simply put, is good for the brain and good for the heart [4, 5]. But it does so much more!
Think of taurine as a tiny superhero that does all kinds of cool things in the body:
- promotes healthy gamma-aminobutyric acid (GABA) levels
- increases nitric oxide
- helps eliminate toxins
- scavenges and neutralizes hypochlorous acid, a reactive species produced by neutrophils
- prevents mitochondrial membrane permeability
- protects neurons from excitotoxicity
- restores fatty acid oxidation and therefore helps generate adenosine triphosphate, and more [6]!
This all means that taurine can help relieve anxiety, promote sleep, increase exercise performance, improve metabolism, and the list goes on. So exciting!
Although taurine can be synthesized in the body from cysteine, the de novo pathways may not always supply sufficient taurine for all its broad functions; therefore, taurine should be obtained from diet. Taurine is found in most meats and fish, and increasing intake of these foods may help restore normal taurine levels. Additionally, taurine supplementation is considered safe and can be tolerated up to 3 g taurine per day without adverse effects.
Tryptophan
Tryptophan, undeniably dubbed as the post-Thanksgiving dinner slumber-inducing molecule, serves as the precursor to serotonin and thus, melatonin—so it is important for mood, sleep, gut motility, and many other important processes in the body. Tryptophan originates in diet—high tryptophan foods include chocolate, meat, tofu, fish, beans, milk, nuts, seeds, oatmeal, and eggs. The recommended daily intake for tryptophan is 4 mg per kilogram of body weight or 1.8 mg per pound, and depending on diet, a person may or may not be getting enough tryptophan. It is important to get sufficient tryptophan specifically because tryptophan spills down two major biosynthetic pathways relevant to the inflammatory neuropsychiatric interface: the generation of the neurotransmitter serotonin as mentioned above, and the formation of kynurenine derivatives and therefore niacin (vitamin B3).
Tryptophan supplementation, however, comes with a caveat and must be approached with caution. In the presence of physiological events that trigger inflammation, high amounts of tryptophan can be directed down the kynurenine pathway and utilized to make excess of excitotoxic molecules (see the following section on kynurenines) [7].
Kynurenines
ZRT Laboratory is now offering testing for the family of tryptophan metabolites collectively termed kynurenines. While tryptophan is the precursor to serotonin and melatonin, upwards of 90% of all tryptophan is diverted down the kynurenine pathway, which is best recognized for its role in inflammatory and anti-inflammatory pathways associated with disease, neuroprotection and neurotoxicity [8]. Under normal physiological conditions, this pathway culminates in generating (niacin-derived) nicotinamide adenine dinucleotide (NAD), a molecule critical to providing the cell with a source of energy.
During inflammatory insults that increase with aging, however, the kynurenine pathway is upregulated—the enzymes tryptophan dioxygenase (TDO) and indoleamine 2,3-dioxygenase (IDO) respond to immune activation by interferons, cytokines, and/or free radicals to generate higher levels of toxic kynurenine metabolites that are neurotoxic and related to brain diseases and mental health [9].
Neuroinflammation
Clinical science started to explore the relationship between inflammation and mental health fairly recently, and the results have been very compelling [10-13]! Sustained activation of the peripheral immune/inflammatory response (e.g., during chronic infections, autoimmune disease, or even cancer), bluntly speaking, is bad for the brain [14]. Vulnerable individuals, even without prior history of mental disorders, can develop mood pathologies as a consequence of being exposed to systemic inflammatory events [15]. Turns out, the brain is not so “immune-privileged” as previously regarded, and the immune-to-brain communication network ensures that. Prolonged inflammation events in the periphery can signal to the brain—either by having cytokines cross the BBB or circumvent the BBB all together—to eventually culminate in microglial activation in the brain, that then results in propagation of pro-inflammatory cytokines locally in the brain. So in a sense, the brain takes a “snapshot” of the peripheral immune response and replicates it with similar molecular components [16].
Connection to Kynurenines
The ins and outs of this mechanism for neuroinflammation-triggering-turbulent-changes-to-one’s-mood phenomenon remains largely uncharacterized, but several pathways have emerged as promising candidates for therapeutic intervention. One such pathway is the kynurenine pathway.
As mentioned above, increased kynurenine levels, in response to elevated TDO/IDO activity during systemic inflammation, lead to increased levels of downstream metabolites with neuroactive properties, both neuroprotective (kynurenic acid) and neurotoxic (3-Hydroxykynurenine or 3-OH Kynurenine). These compounds can inhibit (kynurenic acid) or activate (3-OH Kynurenine) N-methyl-d-aspartate (NMDA) glutamate receptors [17]. Neurotoxicity elicited by 3-OH Kynurenine appears to also be related to generation of oxidative stress produced by reactive radical species, formed as a result of autooxidation. 3-OH Kynurenine gives rise to neurotoxic metabolites, such as quinolinic acid, which also activate the NMDA receptor, induce lipid peroxidation, and promote oxidative stress [18]. 3-OH Kynurenine formed in the periphery and circulating in the bloodstream can easily cross the BBB to induce oxidative stress locally within the brain [19]. Moreover, concurrent serotonin biosynthesis is reduced due to less tryptophan availability—also a factor in compromising brain and consequently mental health [9].
Immune activation of the kynurenine pathway has been reported in anxiety [20], depressive [21], and other [22] brain disorders, including neurodegenerative diseases of aging [23-25].
Xanthurenic Acid
Xanthurenic acid is a metabolite of 3-Hydroxykynurenine that serves as an indirect marker of vitamin B6 status as vitamin B6 insufficiency leads to elevated levels of xanthurenic acid in urine [27]. This pathway is best tested as a loading test following tryptophan ingestion (2 g oral), which results in high xanthurenic acid under situations of vitamin B6 deficiency. If xanthurenic acid levels are elevated in the absence of tryptophan administration, vitamin B6 deficiency is considered to be significant.
Vitamin B6 deficiency that contributes to elevated xanthurenic acid levels can also increase oxidative stress in the body. The hydroxylated quinoline structure of xanthurenic acid can bind iron and increase DNA oxidative damage [28]. (It is fair to mention that other research studies report that when xanthurenic acid is complexed with iron, it can have antioxidant activity [29]. Therefore, it’s hard to say whether xanthurenic acid is definitively prooxidant or antioxidant, and probably depends on the redox state of the cell.) Nevertheless, elevated xanthurenic acid levels may suggest antioxidant insufficiency and ought to be addressed therapeutically.
Histidine & N-Methylhistamine
The original Neuro Advanced profile offered testing for histamine, which proved to be useful in identifying patients with allergies. The newly expanded profile now includes histamine’s precursor, histidine, and metabolite, N-methylhistamine.
Histidine is a semi-essential amino acid that gives rise to the neurotransmitter histamine. Histidine protects neurons, assists with making new blood cells, reduces inflammation and oxidative stress, and helps with tissue repair and growth. Histidine also helps ameliorate fatigue, promotes clear thinking and concentration, reduces appetite, decreases anxiety, and improves sleep and glucose homeostasis. Dosages of histidine up to 4 g/day have shown no negative side effects and have been associated with general improvements. Meat, fish, eggs, soy, and beans are all high in histidine.
N-methylhistamine is a major metabolite of the neurotransmitter histamine, which prominently appears in studies involving gastrointestinal food allergies [30], irritable bowel disease [31], colitis, [32] and others.
Glutamine
Glutamine is an essential and the most abundant free amino acid in the human body. Glutamine provides fuel for rapidly dividing cells (lymphocytes, enterocytes, and epithelial cells of the intestines), helps balance ammonia levels in the body, improves immune system function, contributes to biosynthesis of proteins, amino acids, nucleic acids, and glutathione, and protects intestinal lining. Additionally, glutamine increases glutamate and GABA levels in the brain and in the body.
Although the body usually makes enough glutamine to meet all its needs, extreme stress (e.g. strenuous exercise, persistent stress, or injury) can increase the demand for glutamine beyond the amount naturally manufactured. Low glutamine levels are reported after intense exercise [33], in overtraining syndrome [34], diabetes [35], depression [36], autism spectrum disorder [37, 38], and are associated with high oxidative stress [39]. Research on high glutamine levels is scarce, however high-circulating have been reported to be associated with bipolar depression [40].
Tyrosine & Tyramine
Last, but not least, tyrosine and its metabolite tyramine are now nestled in the “excitatory neurotransmitter” section. Tyrosine is an amino acid obtained from diet (sesame seeds, cheese, soy, meat, nuts, and fish) or synthesized in the body from the amino acid phenylalanine.
Tyrosine gives rise to dopamine, norepinephrine and epinephrine—our favourite motivation/pleasure and stress molecules, thyroid hormones, and the trace amine tyramine. Tyramine is found naturally in food, specifically in aged, fermented, cured, or spoiled food where microbes with decarboxylase enzymes convert tyrosine to tyramine. These foods include aged cheeses, smoked fish, cured meats, some types of beer, red wine, and sherry. In sensitive individuals, high tyramine ingestion can trigger migraines [41]. If your face flushes and you get a splitting headache after ingesting these types of foods/drinks, it is probably your body’s reaction to tyramine. This is because tyramine has vasoactive properties and can increase blood pressure by hijacking sympathetic nerves and displacing norepinephrine.
Laboratory Testing for Neurotransmitters and Amino Acid Precursors
If your patients are suffering from mental health symptoms, and neurotransmitter/amino acid imbalances are suspect, you can get some insight into what might be causing the problems. Much of this information can be obtained from ZRT’s Urine Neurotransmitter Testing. Urine is by collected on a filter strip at home four times throughout the day from morning on awakening to just before bed. The neurotransmitter profile will tell you what neurotransmitters are “off” to help identify the root cause of dysfunction and help develop a suitable and personalized treatment plan that hopefully will help reinstate optimal health.
As your laboratory of choice, we are committed to cutting-edge, innovative, evidence-based testing! Discover a new way to approach mental health in your practice!
Related Sources
References
1. Lucas G. Gut thinking: the gut microbiome and mental health beyond the head. Microb Ecol Health Dis. 2018;29(2):1548250.
2. Guidolin D, Anderlini D, Marcoli M, et al. A new integrative theory of brain-body-ecosystem medicine: from the hippocratic holistic view of medicine to our modern society. Int J Environ Res Public Health. 2019;16(17):3136.
3. Kennedy PJ, Cryan JF, Dinan TG, et al. Kynurenine pathway metabolism and the microbiota-gut-brain axis. Neuropharmacology. 2017;112(Pt B):399-412.
4. Bhat MA, Ahmad K, Khan MSA, et al. Expedition into taurine biology: structural insights and therapeutic perspective of taurine in neurodegenerative diseases. Biomolecules. 2020;10(6):863.
5. Sun Q, Wang B, Li Y, et al. Taurine supplementation lowers blood pressure and improves vascular function in prehypertension: randomized, double-blind, placebo-controlled study. Hypertension. 2016;67(3):541-549.
6. Schaffer S, Kim HW. Effects and mechanisms of taurine as a therapeutic agent. Biomol Ther (Seoul). 2018;26(3):225-241.
7. Tanaka M, Toldi J, Vecsei L. Exploring the etiological links behind neurodegenerative diseases: inflammatory cytokines and bioactive kynurenines. Int J Mol Sci. 2020;21(7):2431.
8. Savitz J. The kynurenine pathway: a finger in every pie. Mol Psychiatry. 2019;25(1):131-147.
9. Suneson K, Lindahl J, Hårsmar SC, et al. Inflammatory depression-mechanisms and non-pharmacological interventions. Int J Mol Sci. 2021;22(4):1640.
10. Lee CH, Giuliani F. The role of inflammation in depression and fatigue. Front Immunol. 2019;10:1696.
11. Petra AI, Panagiotidou S, Hatziagelaki E, et al. Gut-microbiota-brain axis and its effect on neuropsychiatric disorders with suspected immune dysregulation. Clin Ther. 2015;37(5):984-995.
12. Martin-Subero M, Anderson G, Kanchanatawan B, et al. Comorbidity between depression and inflammatory bowel disease explained by immune-inflammatory, oxidative, and nitrosative stress; tryptophan catabolite; and gut-brain pathways. CNS Spectr. 2016;21(2):184-198.
13. D'Mello C, Swain MG. Immune-to-brain communication pathways in inflammation-associated sickness and depression. Curr Top Behav Neurosci. 2017;31:73-94.
14. Dantzer R, Kelley KW. Twenty years of research on cytokine-induced sickness behavior. Brain Behav Immun. 2007;21(2):153-160.
15. Dantzer R, O'Connor JC, Freund GG, et al. From inflammation to sickness and depression: when the immune system subjugates the brain. Nat Rev Neurosci. 2008;9(1):46-56.
16. Dantzer R. From stress sensitization to microglial priming and vice versa: a new era of research in biological psychiatry. Biol Psychiatry. 2019;85(8)619-620.
17. Schwarcz R, Stone TW. The kynurenine pathway and the brain: challenges, controversies and promises. Neuropharmacology. 2017;112(Pt B):237-247.
18. Chiarugi A, Meli E, Moroni F. Similarities and differences in the neuronal death processes activated by 3OH-kynurenine and quinolinic acid. J Neurochem. 2001;77(5):1310-1318.
19. Curto M, Lionetto L, Negro A, et al. Altered serum levels of kynurenine metabolites in patients affected by cluster headache. J Headache Pain. 2015;17(1):27.
20. Kim YK, Jeon SW. Neuroinflammation and the immune-kynurenine pathway in anxiety disorders. Curr Neuropharmacol. 2018;16(5):574-582.
21. Marx W, McGuinness AJ, Rocks T, et al. The kynurenine pathway in major depressive disorder, bipolar disorder, and schizophrenia: a meta-analysis of 101 studies. Mol Psychiatry. 2020. doi: 10.1038/s41380-020-00951-9. Online ahead of print.
22. Huang YS, Ogbechi J, Clanchy FI, et al. IDO and kynurenine metabolites in peripheral and CNS disorders. Front Immunol. 2020;11:388.
23. Ocampo JR, Huitrón RL, González-Esquivel D, et al. Kynurenines with neuroactive and redox properties: relevance to aging and brain diseases. Oxid Med Cell Longev. 2014;646909.
24. Chatterjee P, Zetterberg H, Goozee K, et al. Plasma neurofilament light chain and amyloid-beta are associated with the kynurenine pathway metabolites in preclinical Alzheimer's disease, J Neuroinflammation. 2019;16(1):186.
25. Schwarz MJ, Guillemin GJ, Teipel SJ, et al. Increased 3-hydroxykynurenine serum concentrations differentiate Alzheimer's disease patients from controls. Eur Arch Psychiatry Clin Neurosci. 2013;263(4): 345-352.
26. Bhat A, Pires AS, Tan V, et al. Effects of sleep deprivation on the tryptophan metabolism. Int J Tryptophan Res. 2020;13:1178646920970902.
27. Chiang EP, Selhub J, Bagley PG, et al. Pyridoxine supplementation corrects vitamin B6 deficiency but does not improve inflammation in patients with rheumatoid arthritis. Arthritis Res Ther. 2005;7(6): R1404-1411.
28. Murakami K, Haneda M, Yoshino M. Prooxidant action of xanthurenic acid and quinoline compounds: role of transition metals in the generation of reactive oxygen species and enhanced formation of 8-hydroxy-2'-deoxyguanosine in DNA. Biometals. 2006;19(4):429-435.
29. Kubicova L, Hadacek F, Bachmann G, et al. Coordination complex formation and redox properties of kynurenic and xanthurenic acid can affect brain tissue homeodynamics. Antioxidants (Basel, Switzerland). 2019;8(10):476.
30. Raithel M, Hagel A, Albrecht H, et al. Excretion of urinary histamine and N-tele methylhistamine in patients with gastrointestinal food allergy compared to non-allergic controls during an unrestricted diet and a hypoallergenic diet. BMC Gastroenterol. 2015;15:41.
31. Winterkamp S, Weidenhiller M, Otte P, et al. Urinary excretion of N-methylhistamine as a marker of disease activity in inflammatory bowel disease. Am J Gastroenterol. 2002;97(12):3071-3077.
32. Schwab D, Hahn EG, Raithel M. Enhanced histamine metabolism: a comparative analysis of collagenous colitis and food allergy with respect to the role of diet and NSAID use. Inflamm Res. 2003; 52(4):142-147.
33. Keast D, Arstein D, Harper W, et al. Depression of plasma glutamine concentration after exercise stress and its possible influence on the immune system. Med J Aust. 1995;162(1):15-18.
34. Rowbottom DG, Keast D, Morton AR. The emerging role of glutamine as an indicator of exercise stress and overtraining. Sports Med. 1996;21(2):80-97.
35. Liu X, Zheng Y, Guasch-Ferré M, et al. High plasma glutamate and low glutamine-to-glutamate ratio are associated with type 2 diabetes: case-cohort study within the PREDIMED trial. Nutri Metab Cardiovasc Dis. 2019;29(10):1040-1049.
36. Umehara H, Numata S, Watanabe SY, et al. Altered KYN/TRP, Gln/Glu, and Met/methionine sulfoxide ratios in the blood plasma of medication-free patients with major depressive disorder. Sci Rep. 2017;7(1):4855.
37. Moreno-Fuenmayor H, Borjas L, Arrieta A, et al. Plasma excitatory amino acids in autism. Invest Clin. 1996;37(2):113-128.
38. Rolf LH, Haarmann FY, Grotemeyer KH, et al. Serotonin and amino acid content in platelets of autistic children. Acta Psychiatr Scand. 1993;87(5):312-316.
39. Pietzner M, Kaul A, Henning AK, et al. Comprehensive metabolic profiling of chronic low-grade inflammation among generally healthy individuals. BMC Medicine. 2017;15(1):210.
40. Pålsson E, Jakobsson J, Södersten K, et al. Markers of glutamate signaling in cerebrospinal fluid and serum from patients with bipolar disorder and healthy controls. Eur Neuropsychopharmacol. 2015;25(1):133-140.
41. Burns C, Kidron A. Biochemistry, Tyramine. In: StatPearls [Internet]. Treasure Island, Fla. StatPearls Publishing. 2021.